RESEARCH
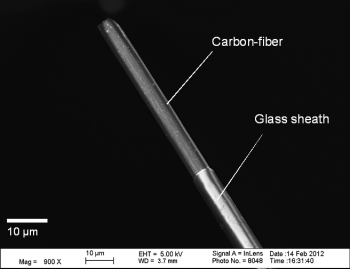
My research group employs fast-scan cyclic voltammetry (FSCV) at carbon-fiber microelectrodes (Fig. 1) in order to investigate millisecond-timescale, intracellular signaling events between neurons and their relationship with behavior. FSCV is useful because it can selectively measure the release of electroactive chemical species, including catecholamines, from cells, tissues, and living animals with high temporal resolution (we typically collect 10 measurements per second). Two primary aims of my research group are to: (1) apply existing analytical techniques toward understanding neurological disease states, including Huntington’s disease and chemobrain and (2) develop new methods that will allow us to obtain new types of measurements from biological systems. A sampling of just a few of our active projects are described in the ensuing paragraphs; however, we also are working on other biologically relevant projects that make use of microfluidics and novel in vivo sensors. If you have questions about these and other exciting opportunities, please feel free to email me.
Huntington’s Disease
Huntington’s disease (HD) is a fatal, neurodegenerative movement disorder characterized by uncontrollable muscle movements and mental illness. HD patients typically die 15 to 20 years following symptom onset. We and others have recently discovered that the release of dopamine, a key neurotransmitter in motor and cognitive signaling, is sharply attenuated in animal models of HD. To understand the contributions of abnormal neurotransmitter release in the debilitating motor symptoms of HD, electrochemical techniques are applied to brain slices as well as in vivo to animal models of HD.
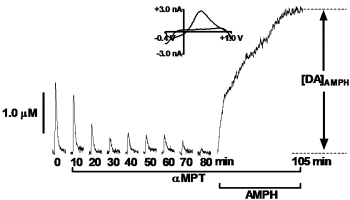
Over the past seven years, our laboratory has characterized dopamine release and uptake properties of several genetically-altered mouse and rat strains that model HD. Interestingly, we have found that dopamine release is impaired in all strains, even though dopamine content is only moderately decreased. One interesting finding is that the dopamine reserve pool, which serves as a storage area for additional neurotransmitter to be used under conditions of high synaptic activity, is diminished in R6/2 mice, the most commonly studied HD model (Fig. 2; Ortiz et al., 2010). Our current efforts are aimed at resolving the impact of other neurotransmitter systems, such as glutamate and GABA, on dopamine release.
Chemobrain
“Chemobrain” is a decline in cognitive function experienced by patients undergoing chemotherapy treatment. Recent studies comparing cognitive function before and after chemotherapy suggest that approximately 20-30% of cancer patients will exhibit lower cognitive performance after chemotherapy than would be expected. Although the phenomenon is not yet completely understood, developing an understanding of chemobrain is becoming more significant as the survival rates of cancers continue to increase. Dopamine is an important neurotransmitter involved in cognition, memory and reward systems in the brain. To determine the impact that chemotherapy has on dopamine release/uptake and reserve pool storage, we are using fast-scan cyclic voltammetry (FSCV) at carbon fiber microelectrodes to measure electrically-evoked dopamine release and uptake in striatal brain slices from male Wistar rats treated with various chemotherapy regimens. Future directions include testing new therapeutic agents and applying novel methodologies to be used in vivo, including microfluidic sampling devices, in order to measure changes in other neurotransmitter systems.
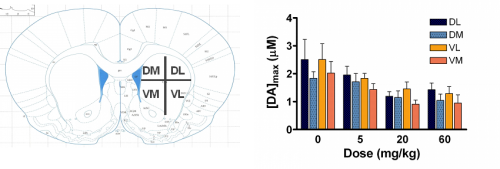
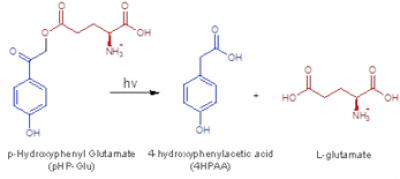
Caged Compounds
Photoremovable protecting groups, or “caged” compounds, provide precise spatial, temporal, and concentration control over the application of biologically active molecules through light-initiated reactions. Recently, our research group has pursued the use of fast-scan cyclic voltammetry (FSCV) at carbon-fiber microelectrodes to measure dopamine release while also monitoring the progress of photoactivation reactions in solution and in brain tissue slices.
We are currently using this technology to investigate interactions between the glutamate, and abundant excitatory neurotransmitter, and dopamine. In collaboration with Prof. Rich Givens, KU Department of Chemistry, we have photoactivated p-hydroxylphenacyl glutamate in brain tissue slices (see Figs. 4 and 5). The development and application of this technology is important because it will allow investigators to probe neurotransmitter interactions on sub-second to millisecond timescales. Thus, this method should have broad applicability to many areas of research that focus on interactions between neurotransmitters, including, but not limited to: (1) fundamental neurobiological studies aimed at decoding relationships between brain dopamine and glutamate/GABA release; (2) investigations specifically addressing dopamine-related movement disorders [e.g. Huntington’s disease (HD), Parkinson’s disease (PD), Tourette’s syndrome (TS)]; (3) studies of addiction and depression; and (4) experiments aimed at resolving the mechanisms of action of CNS-active pharmacological agents.
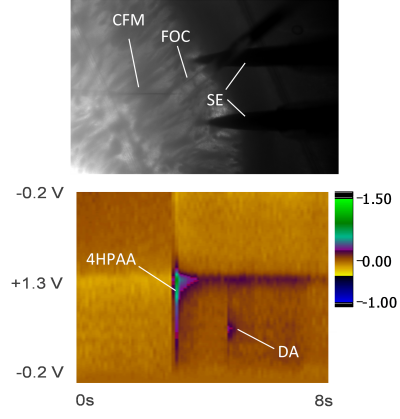